Our research team, lead by Prof. Guillermo Ameer, develops biomaterials and nanotechnology for regenerative engineering, tissue engineering, medical devices, drug delivery, and cell delivery applications. We pioneered the development of citrate-based antioxidant biomaterials referred to as polydiolcitrates. Our research can be classified into two areas: 1) citrate-based elastomers and composites thereof, and 2) citrate-based injectable biomaterials.
Regarding the first area, we and others have demonstrated that citrate-based elastomers and composites can be engineered to have the following properties: a. tunable mechanical and degradation properties, b. antioxidant activity, c. antimicrobial activity, d. photoluminescence, and e. shape memory response. Furthermore, they are easy to synthesize, and scale up. Polydiolcitrates have been investigated for the tissue engineering of blood vessels, cartilage, bone, bladder, and ligament. They are also investigated as part of new strategies to modulate the body’s response to injury (e.g. perivascular devices or bioengineered vascular grafts designed to improve bypass surgeries).
Regarding the second area, citrate-based injectable biomaterials, we are developing water-soluble thermoresponsive oligomers referred to as Nanonets™ as well as photoresponsive liquid polymers. We have demonstrated that Nanonets™: a. have antioxidant activity; b. can be used for drug, protein, gene, and cell entrapment and delivery; c. can be engineered to have photoluminescent properties; and d. can incorporate nanoparticles to create injectable composite materials. Nanonets™ are being investigated for use in wound healing, islet transplantation, bone regeneration, localized delivery of therapeutics, and transarterial embolotherapy to treat arteriovenous malformations and cancer. As to our work in photoresponsive liquid polymers, we have engineered liquid polymers that can be cured within seconds to form a tough elastomeric material. Potential applications include laser-based stenting with drug-eluting biodegradable elastomers for the treatment of atherosclerosis and 3D printing of patient-specific medical devices.
Current research ongoing in the lab (Oct. 2017) Click to zoom.
Multifunctional Biomaterials
By using the basic chemistry of ester bonds, novel functional materials have been synthesized based on citric acid, a tri-functional natural monomer, and aliphatic diols of different length. These polyesters, referred to as polydiolcitrates (PDCs), may display elastomeric or thermoresponsive properties and exhibit intrinsic antioxidant properties. They allow for further functionalization and customizable mechanical properties and degradation times.
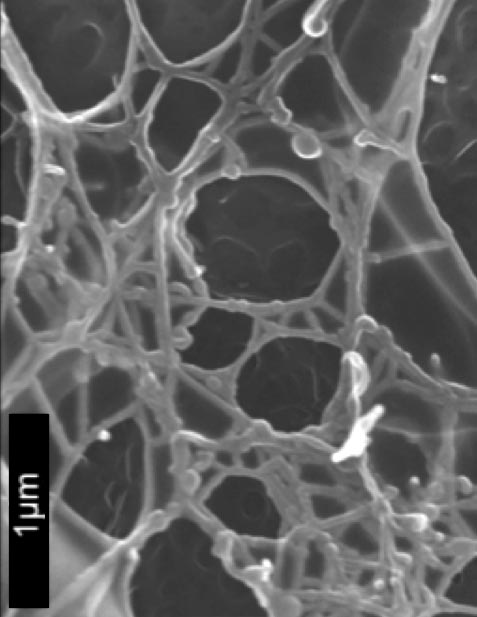
SEM of polydiolcitrate hydrogel nanofibers.
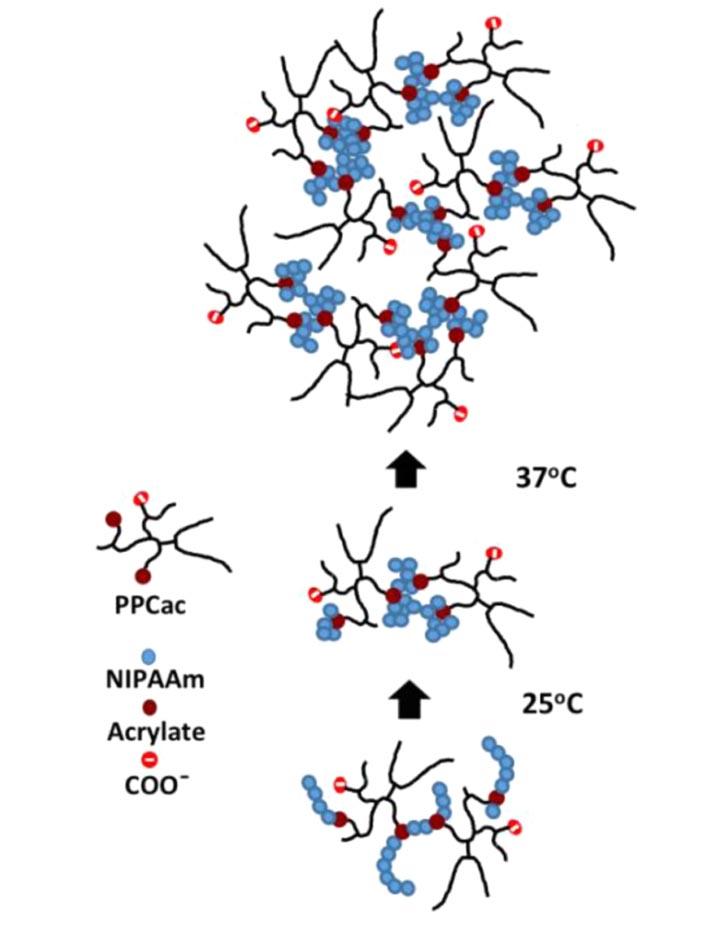
Mechanism of thermoresponsive hydrogel transition.
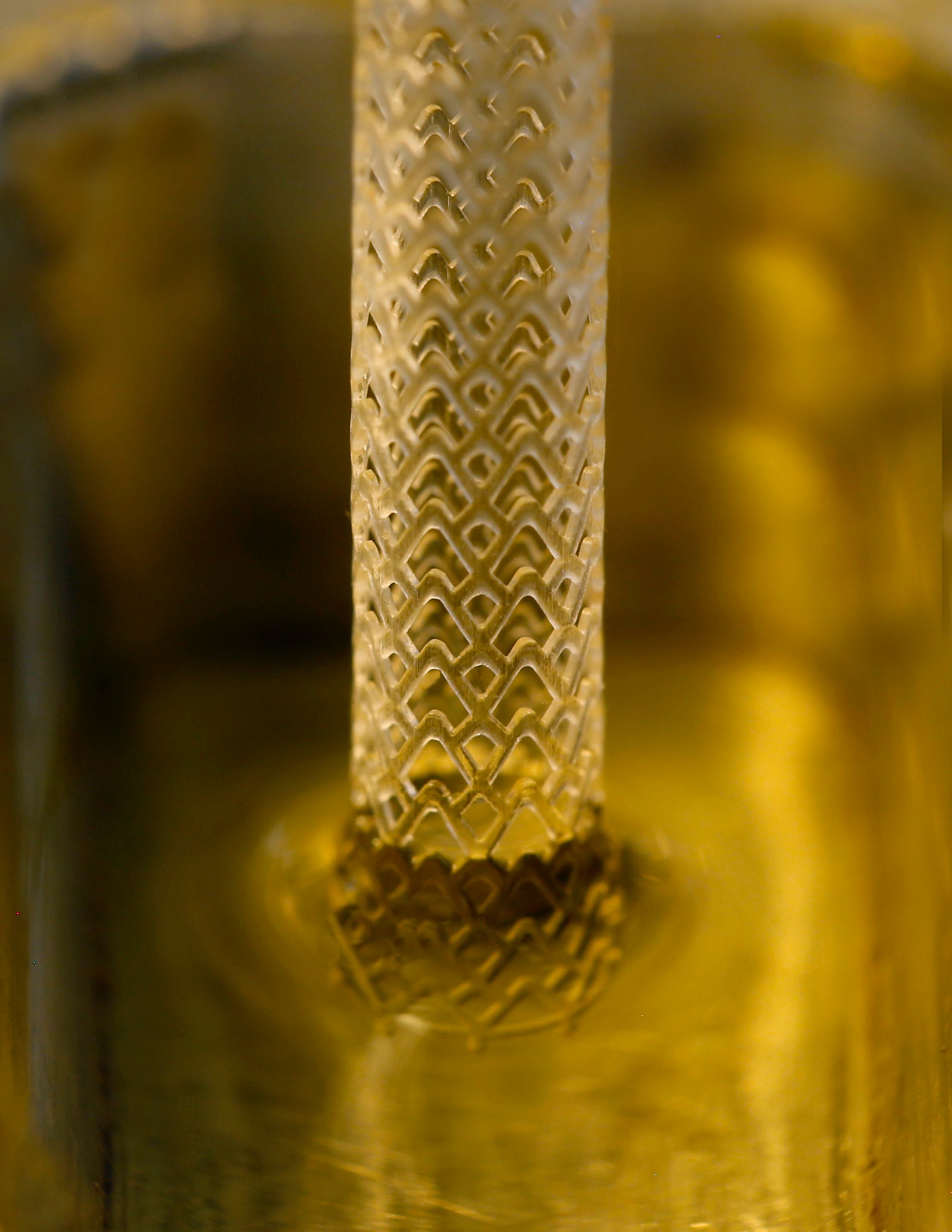
Light-induced crosslinking is utilized to polymerize a citric acid-based stent via 3D printing.
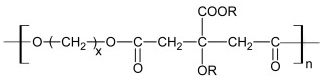
General formula of polydiolcitrates, a biodegradable, non-toxic, elastomeric class of polymers developed in our laboratory.
UV-initiated 3D printing of a vascular stent from biomaterial “ink.”
(Jump to 40s—5:30m in the timestamp—for stent emergence.)
Some of the approaches currently under study are:
- PDC scaffolds for vascular tissue engineering.
- PDC composites for bone tissue engineering.
- PDC scaffolds for pancreatic islet transplantation.
- UV-curable polymers for 3D printing of implantable devices.
- Influence of cell function and behavior via PDC micro-/nano-patterning.
- Thermoresponsive, injectable PDCs as a wound dressing; cell, drug, or gene delivery platform; or embolic agent.
Surface Engineering to Control Cell and Tissue Responses
Xinlong Wang
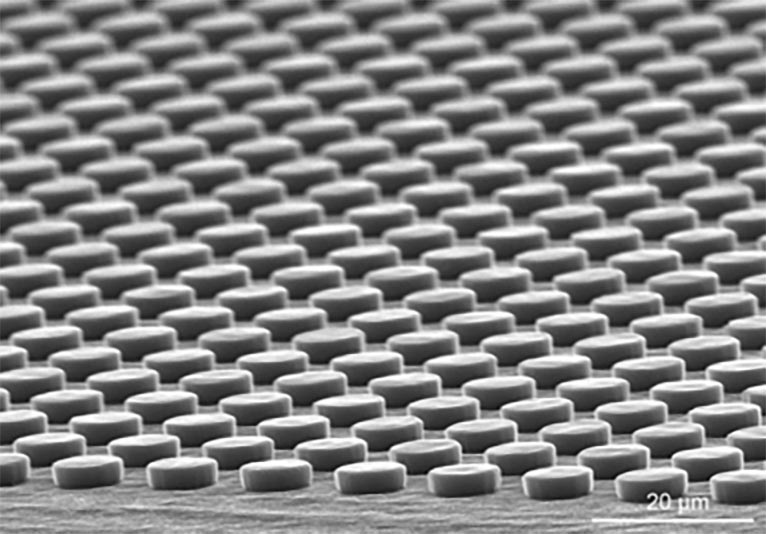
Micro-patterned surface.
Micro- and nano-patterning have been widely applied to mimic cell niche for manipulation of cell functions. Using the citric acid-based polymers developed in the lab—poly(1,8-octanediol-co-citrate) (POC), methacrylated poly(1,8-octanediol-co-citrate) (mPOC), etc.—and micro-/nano-imprinting methods, we are able to prepare the patterned surfaces with various micro- and nano-features. The patterned substrates affect cell morphogenesis and many other cell functions. Meanwhile, the biodegradable and biocompatible patterned POC substrates show the potential to be implanted in vivo to facilitate tissue formation.
Modeling Stimuli-Responsive Hydrogels
Simona Morochnik, in collaboration with Szleifer Group
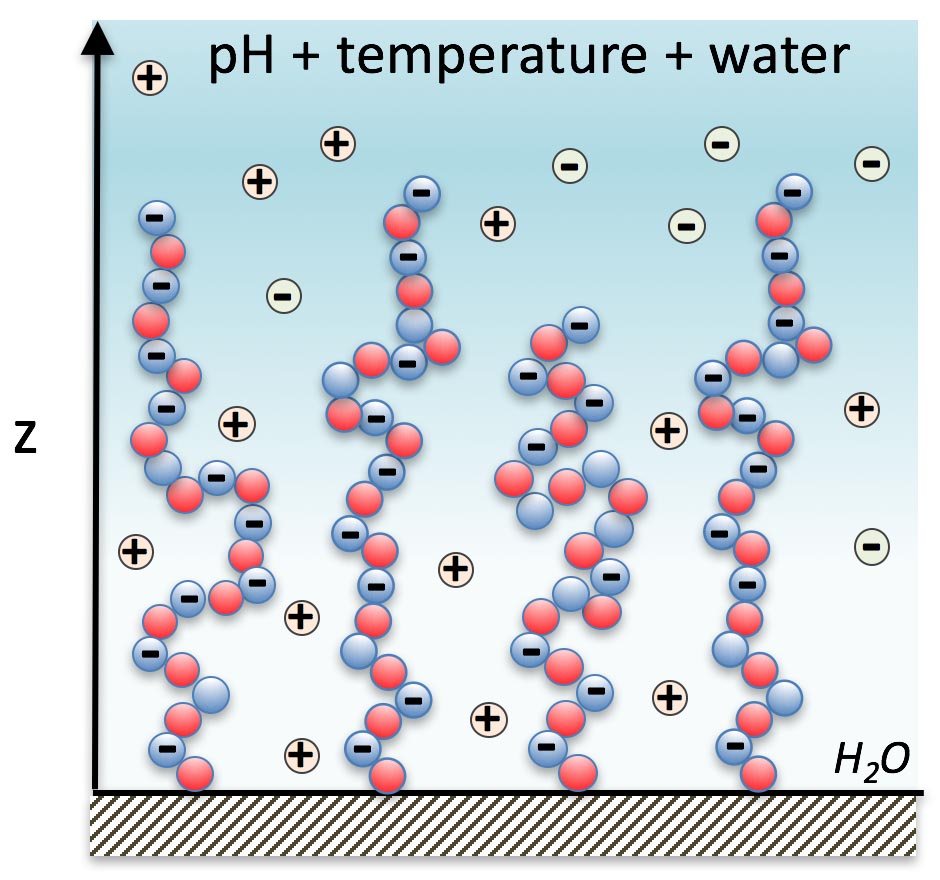
Schematic of the responsive tethered layer model.
Today, there is limited understanding of thermoresponsive materials at the molecular level. Namely, it is not clear how small changes in the molecular architecture will change the physical and chemical response of the material to different stimuli, e.g. temperature and pH changes. Because poly(polyethylene glycol citrate-co-N-isopropylacrylamide (PPCN) gels at physiological temperature, it has the potential to be an injectable, vascularizing innovative bone material. To optimize the properties of the gel as a function of its molecular composition, we use molecular theory to predict the thermodynamic and structural properties of PPCN.
Preliminary experimental observations show that the gel transition temperature can change based on different peptide functionalization, hydrophobicity and other chemical conjugations. This is where molecular theory plays a key role. Liquid-gel transitions are essential to understand and control in order to design smart materials that will support functionalization, entrap cells and allow for slow release of therapeutic molecules. In order to do this systematically, we are collaborating with the Szleifer lab to develop computational tools that will explain our experimental observations and provide deep understanding of the relationship between molecular organization, physical interactions and chemical states in our material.
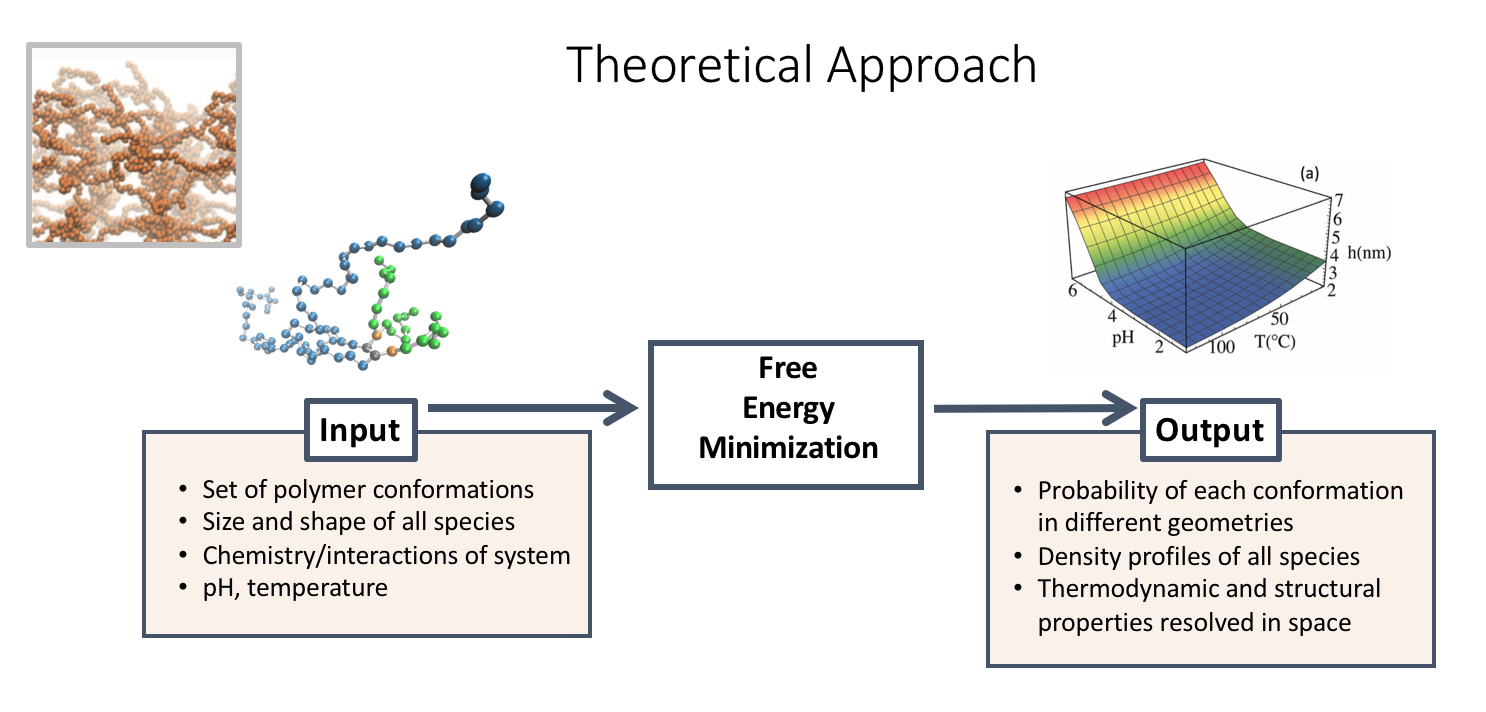
To model the effect of the competitive relationship of temperature and pH on phase behavior, we can visualize both parameters as a 3D graph of the average height profile.
Stem Cell Engineering
Bin Jiang, Jimmy Su, Xinlong Wang, and Nancy Rivera Bolaños
Induced pluripotent stem (iPS) cells have the ability to differentiate into any cell type in the body, which makes them an ideal source for a variety of cell-based tissue engineering applications. One advantage to iPS cells is their potential to be induced from patient autologous cells for stem cell therapy of various pathologies; however, disease progression negatively impacts the robustness of cell growth for in vitro manipulation and induction efficacy. We are therefore developing methods to induce pluripotency in cells collected from target patients of stem cell therapy.
Additionally, one major safety concern of stem cell therapy is the tendency of iPS cells to spontaneously form teratomas—a certain type of tumors—upon implantation. Therefore, complete differentiation of these cells must be carried out in vitro under controlled conditions in order to produce a high yield of terminally differentiated cells prior to implantation. We are also working to develop differentiation protocols for our patient-derived iPS cells.
Successful completion of these studies would allow us to design stem-cell niche directed therapies aimed at maximizing the yield of lineage-specific cells while maintaining low immunogenicity. The long-term goal of this project is to direct the differentiation of iPS cells in order to produce functional autologous vascular cells for cell-based tissue-engineering therapies. Accomplishing this goal will contribute toward establishing vascular cell sources that can be used to improve current treatments of cardiovascular disease.
Regenerative Engineering
Regenerative engineering is the convergence of advanced materials science, stem cell and developmental biology, physical sciences, and clinical implementation to solve challenges in tissue and organ function and regeneration. In this area, we are currently pursuing the following main avenues:
Vascular Engineering
Bin Jiang, Jimmy Su
Cardiovascular disease is the number one killer in the USA and vascular disease is a significant contributor to the number of deaths. We are interested in designing and evaluating biodegradable materials that would be conducive to the formation of small-diameter blood vessels. An ideal engineered vascular graft should be non-thrombogenic, non-toxic, and have viscoelastic properties that are similar to those of the native host vessel it will replace. Several laboratories have been investigating various scaffold materials and cell sources in an attempt to meet these requirements. Our lab is interested in a) the characterization and use of progenitor cells from blood for tissue generation, and b) the effect of the mechanical properties of the biomaterial scaffold on cell signaling and tissue generation.
Many approaches to optimizing vascular grafts require the creation of a functional endothelial cell monolayer within the lumen of the graft. This requirement has prompted significant research into the use of adult stem cells differentiated into endothelial-like cells to cover the scaffold. One potential source of adult stem cells is endothelial progenitor cells (EPCs), found in circulating peripheral blood. There are few studies on the interactions of these cells with biomaterials for potential applications in vascular tissue engineering; specifically, the engineering of a functional endothelium.
Our lab is also interested in modifying the material properties of vascular graft scaffolds in order to improve graft function over long timecourses. With these modifications we aim to reduce the rate and extent of graft restenosis, scarring, and calcification, and improve the prognosis of long-term stent implantation in sclerotic and occluded vessels.
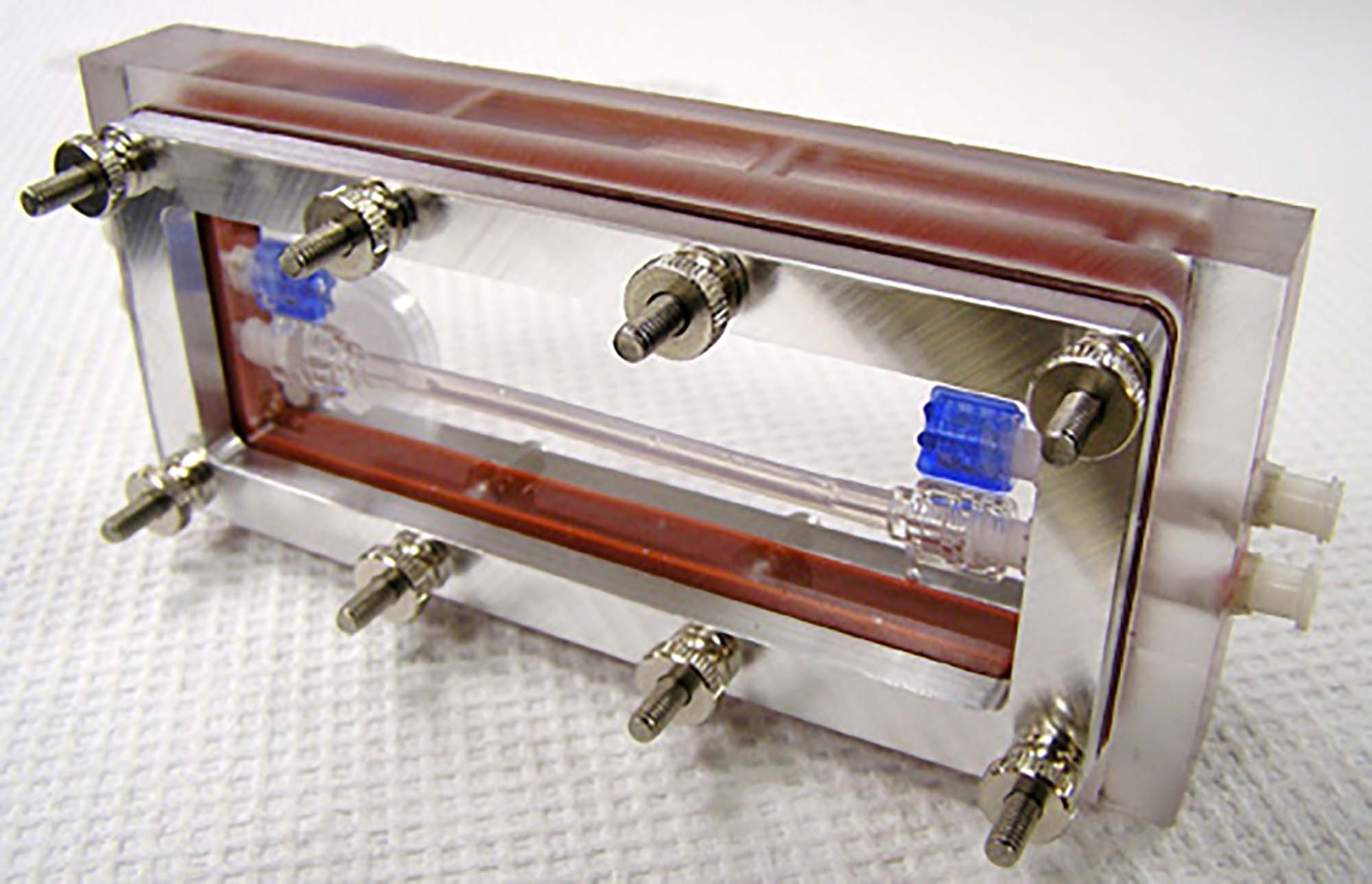
Bioreactor developed in our lab for the in vitro growth and monitoring of blood vessels under dynamic culture conditions.
Orthopaedic Engineering
Samantha Huddleston
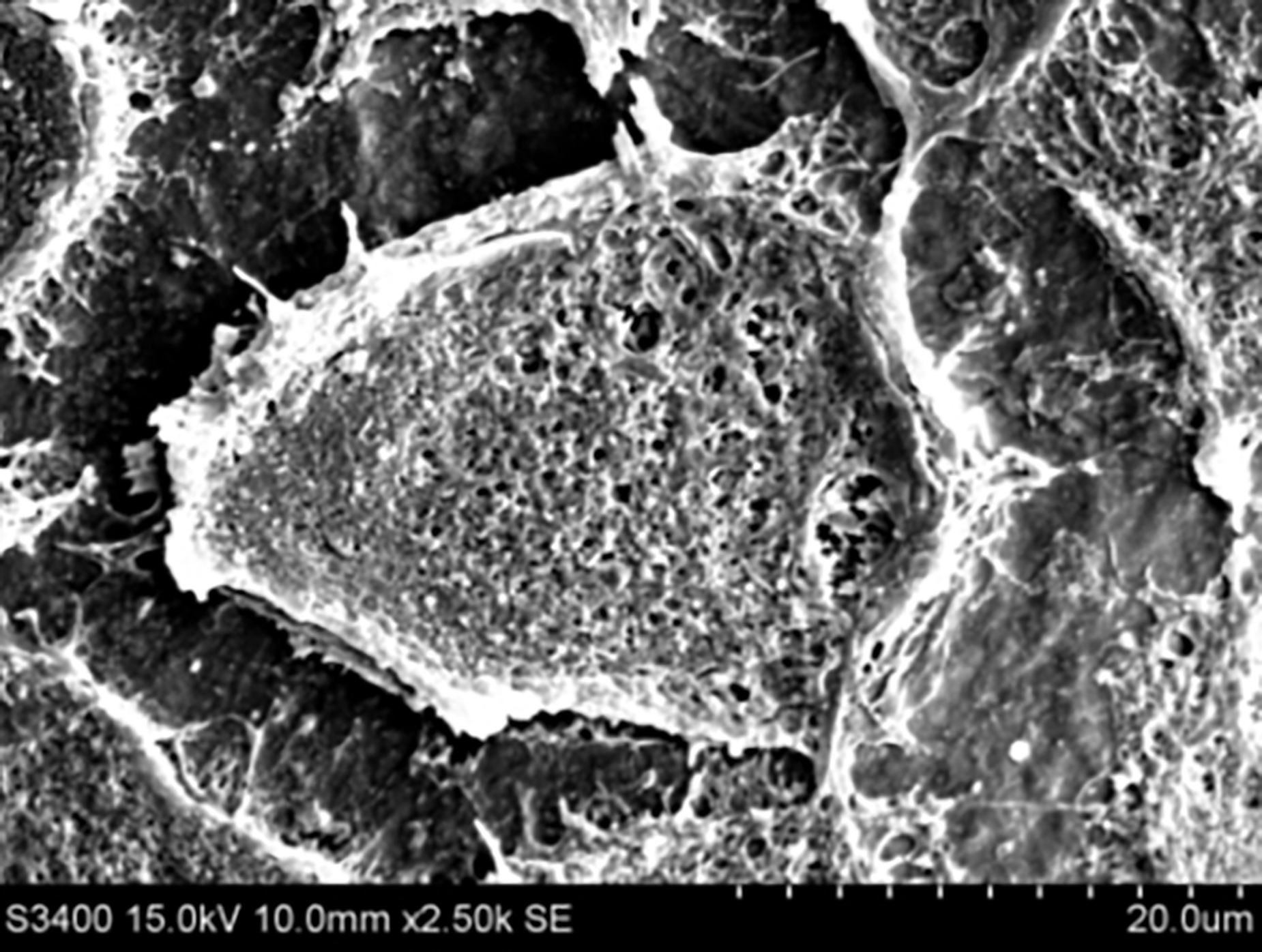
A mesenchymal stem cell on a poly(octanediol-co-citrate)-hydroxyapatite composite.
Orthopaedic research is an exciting field that is also in high demand due to the growing elderly population as well as the rise in sports-related injuries. We are specifically addressing problems associated with bone defects and injuries to the ligaments. The addition of hydroxyapatite (HA) and other bioceramics to our PDC polymers forms composites that suit the mechanical and degradation properties for bone and ligament tissue engineering, or are capable of promoting bone formation via molecular induction. For our bone defect projects, our goal is to create a material that is osteoconductive and osteoinductive and can initially restore a bone defect, but eventually be replaced by the host’s tissue over time. Using material science, molecular biology, and clinical prinicipals, mesenchymal stem cells are used as the cell source to engineer biomaterial- and cell-based bone graft. Gene delivery and drug elution from PDC hydrogels are also potential media through which biomaterials may support osteoregeneration, and are currently being explored as well.
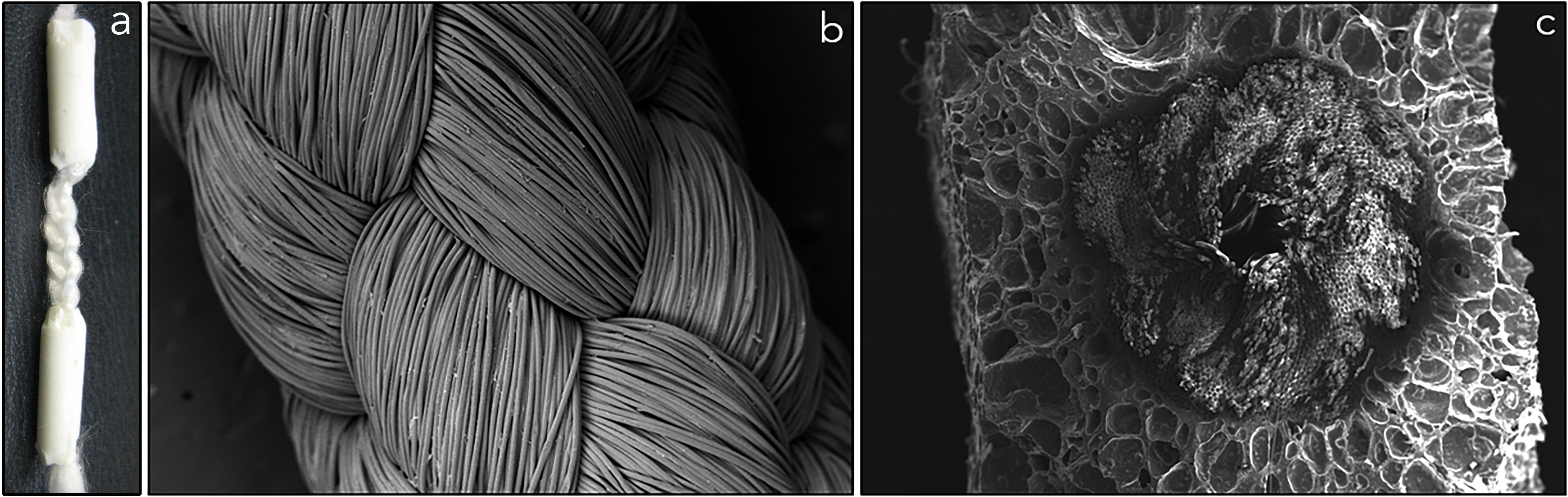
a) Biodegradable, tri-component graft for ACL reconstruction. b) Braided poly(L-lactic acid) (PLLA) intraarticular graft. c) Cross-section of POC-HA wrapped around PLLA graft at bony ends.
Islet Transplantation Engineering
Jacqueline Burke, in collaboration with Scott Group;
In the treatment of Type I diabetes, pancreatic islet transplation has been developed to reduce dependence on exogenous insulin injections. However, current transplantation procedures suffer from a lack of anti-inflammatory, resorbable biomaterials scaffolds; insufficient cell sources of islets; and poor long-term efficacy of grafted cells. We aim to address these concerns by engineering our PDC scaffolds to facilitate islet delivery and enhance islet survival and function post-operatively.
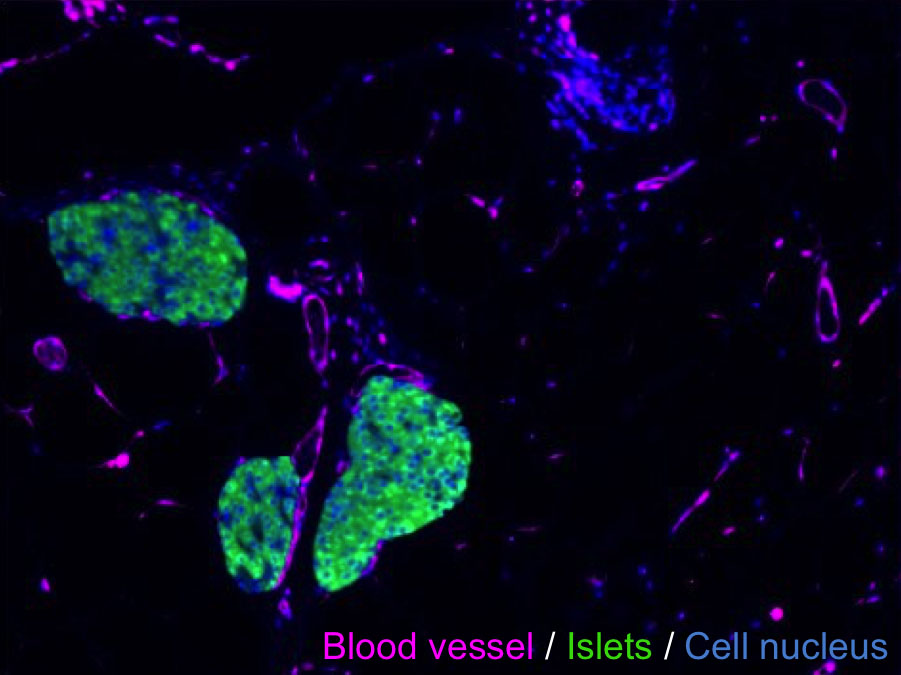
Regenerated pancreas within the mouse epididymal fat pad.
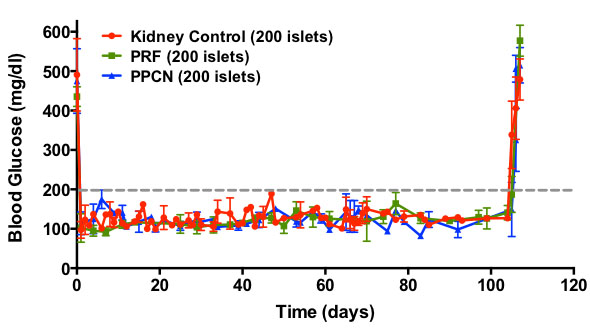
Demonstrated enhancement of insulin-mediated glucose-responsiveness due to graft transplantation, with removal of this effect upon graft excision.
Wound Engineering
Joseph Song
Chronic wounds such as non-healing diabetic foot ulcers continue to be a great challenge for the physician and contribute to increasing healthcare costs. Numerous factors have been reported to contribute to chronic wound healing, including diminished peripheral blood flow and decreased local neovascularization, retarded recruitment of endothelial cells and fibroblasts, delayed collagen deposition, and rampant oxidative stress. We use thermo-responsive hydrogels developed in our lab to deliver genes, proteins, or metal organic frameworks to diabetic wounds with the purpose of promoting angiogenesis, cellular migration, and collagen deposition; and/or reducing reactive oxygen species, thus improving diabetes-impaired wound healing.
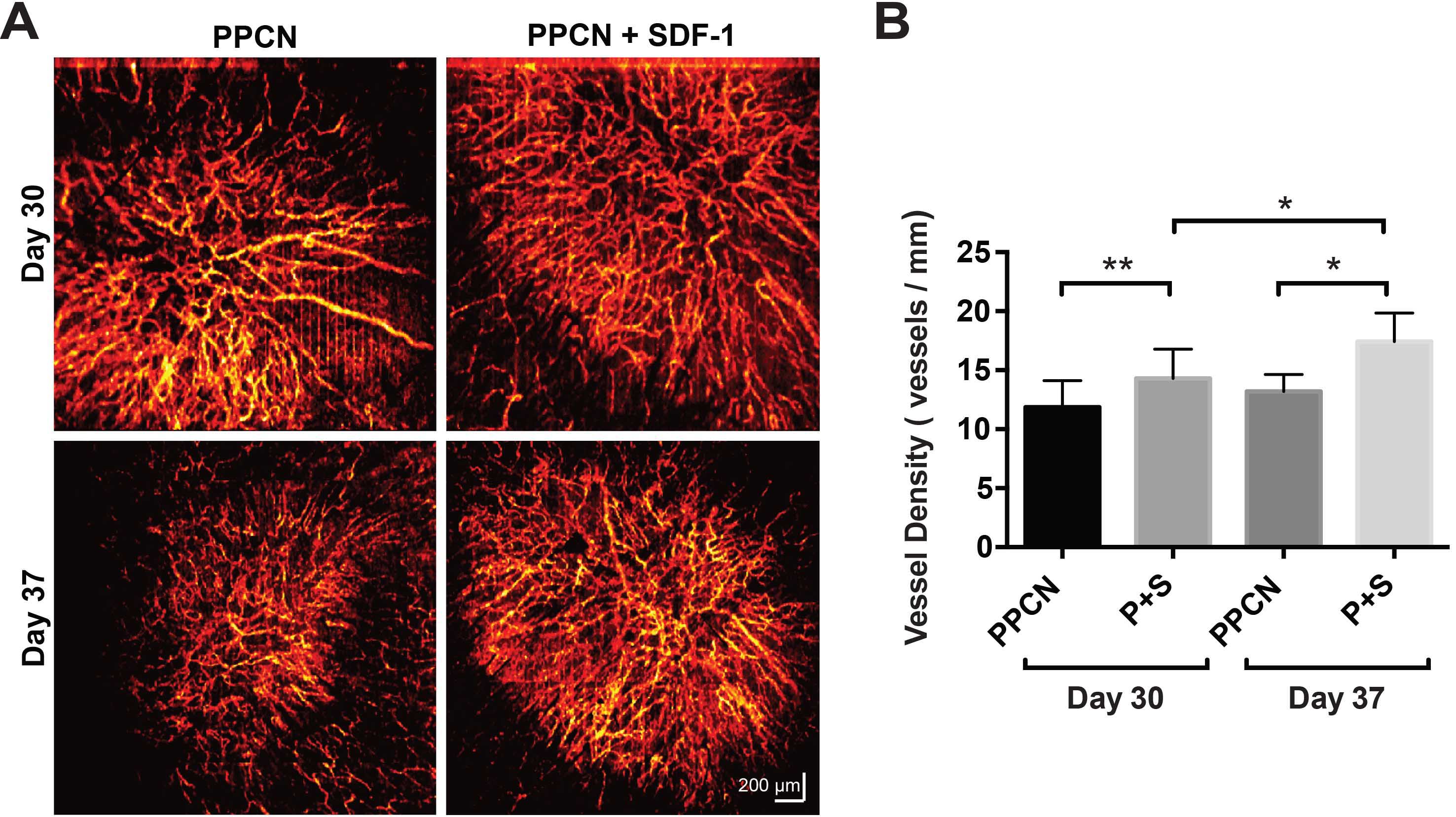
A) Wound microangiography of diabetic wounds treated with hydrogels, displaying sustained greater vascularization in wounds treated with protein-releasing hydrogels (PPCN+SDF-1). B) Vessel density in the wound region.
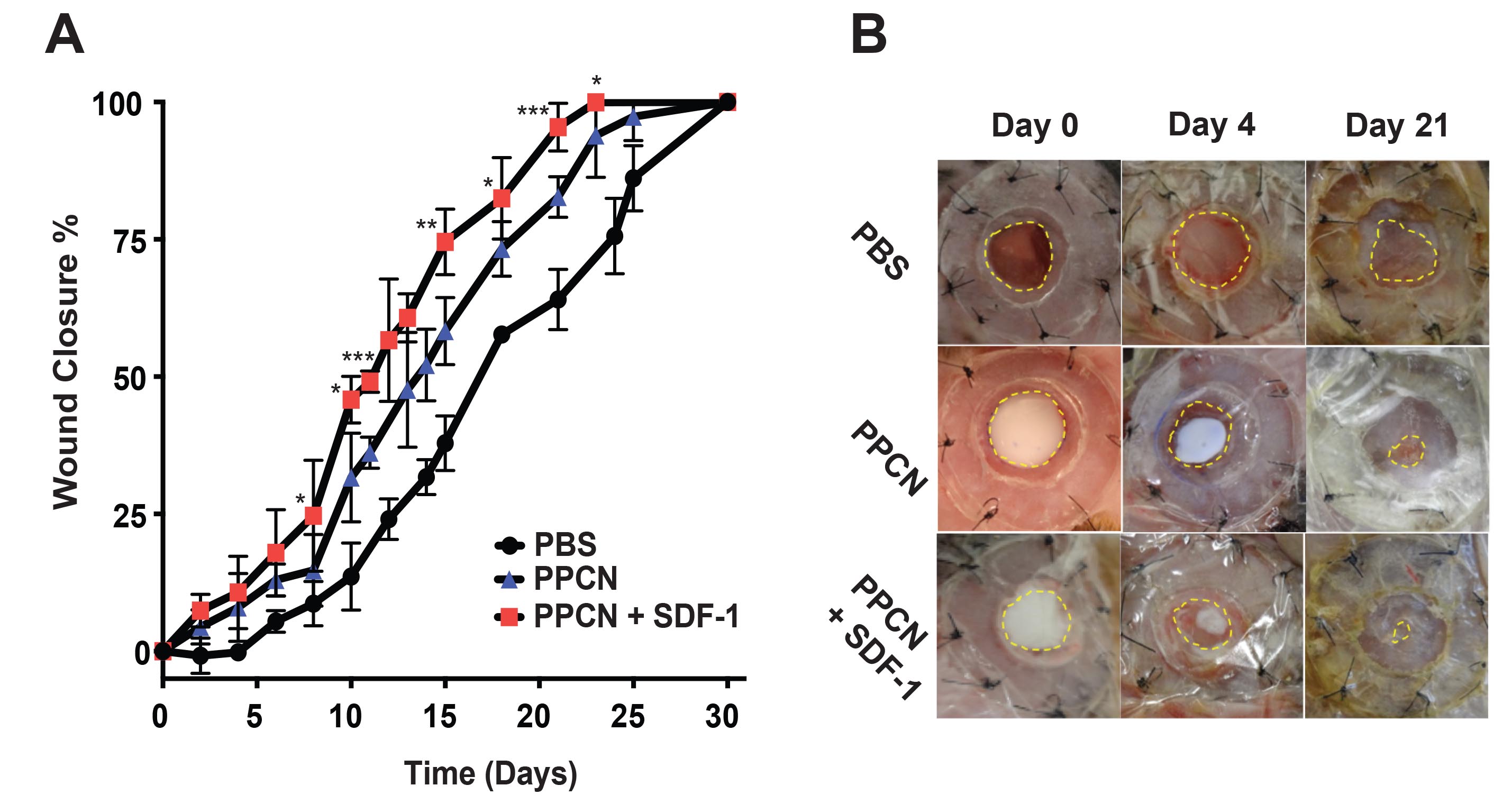
Quantification (A) and images (B) of diabetic wound closure for wounds treated with hydrogel (PPCN) or protein-releasing hydrogel (PPCN+SDF-1).